SSDriver
Senior Member
- Location
- California
- Occupation
- Electrician
I don’t see any reference to the EMT conduit in this table.
As calculated the short-circuit it is 4 times the device rating, if the distance from source up to fault point it is not more than it is noted, indeed.
However, the voltage drop it is not 40V [ for any row].
If the circuit breaker delay setting it is 1 sec or less according to IEC/TS 60479_1 standard 120 V touch voltage is permissible.
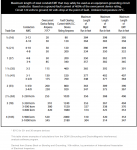
I find it interesting that EMT and IMC have a greater circuit length than Rigid.